INTRODUCTION
The origin of first life was solely depending on environmental condition of primitive Earth. An appropriate site for the origin of first life would require liquid water, source of bioorganic compounds, a source of energy to drive polymerization reactions among bio-monomers. The bio-monomers undergo physical and chemical interactions on appropriate surface to form bio-polymers from which first life originated. During the course of chemical evolution, cyanide ions were abundant in nature. Cyanide ion is smaller in size and is considered as a strong ligand due to the presence of triple bond. It shows basic, ambidentate characteristics and forms a variety of complexes with transition metal ions. Double metal ferrocyanides mostly insoluble in water could have played an important role as adsorbents, ion-exchangers and photosensitizers during the course of chemical evolution on early Earth. Recent investigation suggested that double metal ferrocyanides might have abundantly existed in primitive Earth environment. Platinum and tungsten ferrocyanides were synthesized and characterized by elements and spectral studies. For the generation of life organic compounds are essential. It has been controversial that major sources of organic compounds used for the generation of terrestrial biosphere was of terrestrial origin or of extraterrestrial origin. It has been investigated that bioorganic compounds such as amino acids are easily formed in a mixture of CH4, NH3, and H2O, that is, a ‘strongly’ reduced atmosphere, where ultraviolet light1 and spark discharges2 are used as energy sources. It is also reported that the primitive atmosphere of the earth was composed of CO2, CO, N2 and H2O3, that is, the slightly reduced gases, and that CO is a major carbon compound found in extraterrestrial environments like comets4. It is not easy to form amino acids from these slightly reduced gases by ultraviolet light5 or by electric discharge6),(7. The present experiment indicates that amino acids or their precursors are easily produced even in slightly reduced gases by irradiating with high energy charged particles. Cosmic rays might be an effective energy source for abiotic formation of amino acids and their bioorganic compounds in the primitive atmosphere of the earth as well as other planetary/cometary atmospheres8),(9.
Titus et al.10 studied the adsorption of alanine, phenylalanine, tyrosine and tryptophane on highly hydrophobic NaZSM - 5 zeolite. Adsorption of phenylalanine and tryptophane on non-ionic polymeric sorbents was investigated by Lee et al.11. Zimnitsky et al.12 described multilayer adsorption glycine, L-alanine, L-proline on oxidized cellulose. The surface - enhanced Raman spectroscopic studies of dissociative adsorption of glycine, threonine and serine on platinum and gold electrodes in alkaline solutions were studied by Xiao et al.13. The adsorption of serine, tyrosine and histidine on a gold electrode from 0.1 M LiClO4 aqueous solutions was investigated by Slojkowska et al.14. Vlasova et al.15 reported adsorption of arginine, histidine, lysine and ornithine on the surface of highly dispersed silica from aqueous solution. The solution of L-aspargine, DL-threonine, L-lysine, L-leucine, DL-methioine, L-tyrosine, L-phenylalanine, and DL-tryptophane on the non-polar macroporous adsorbents, and amberlite XAD-2 and XAD - 4 was studied by Doulia et al.16. Wedyan et al.17 investigated isomer - selective adsorption of amino acids by components of natural sediments.
The adsorption of L-valine, L-leucine and L-phenylalanine, bearing different substituted groups was investigated on a Cu (III) electrode by in situ ECSTM as described by Wang et al.18. The geometry of adsorbed glycine and alanine molecules on the intrinsically chiral Cu (3, 1, 17) facets was reported by Rankin et al.19. Basiuk et al.20 investigated the estimation of thermodynamic parameters for amino acid adsorption on silica from water using HPLC technique. The adsorption and desorption dynamic of phenylalanine and tryptophane in a non-adsorbent XAd-16 column was studied by Yang et al.21.
Processes in chemical evolution must have involved several catalysts multifunctional in nature. It is assumed that during the course of chemical evolution, cyanide could have formed some metal ferrocyanides. As these metal ferrocyanides are mostly insoluble in water, they are therefore considered to have settled at the bottom of the primeval ocean or at sea shone. The metal cyanogen complexes might have acted as an important prebiotic catalyst22),(23),(24. A search of literature indicated that very little is known about the interaction of amino acids with metal ferrocyanides. In view of this, attempts were made to study the interaction of amino acids with metal ferrocyanides. The present work describes the adsorption of DL-histidine, DL-threonine and DL-valine on platinum and tungsten ferrocyanides
RESULTS AND DISCUSSION
Effect of pH on the adsorption of amino acids on metal ferrocyanides
The percentage uptake of DL-histidine, DL-threonine and DL-valine as a function of pH was studied over the pH range (1.0 - 10.0). The effect of pH on the adsorption of amino acids on platinum and tungsten ferrocyanides are shown in Figure 1. The percentage uptake of amino acids on metal ferrocyanides were calculated by general formula
The values of percentage adsorption of DL-histidine, DL-threonine and DL-valine on platinum and tungsten ferrocyanides are given in Table 3 & 4 respectively. The following order of uptake was observed with amino acids for the both adsorbents
DL-threonine > DL-valine > DL-histidine
Maximum adsorption in case of all three amino acids takes place near to their corresponding isolectric point. Adsorption of amino acids on metal ferrocyanides could be due to amino acid interaction with replaceable metal cations of platinum and tungsten present outside the coordination sphere of metal ferrocyanides.
At higher pH considerable decrease in adsorption of amino acids on platinum and tungsten ferrocyanides may be because at higher pH coordination of available OH- with metal cations become competitive with that of DL-histidine, DL-threonine and DL-valine molecules. The adsorption of amino acids on metal ferrocyanides may be due to presence of amino group, carboxylic group and hydrophobic carbon chain in amino acids molecule, which acts as site for interaction with metal ferrocyanides surface.
Effect of concentration for adsorption of amino acids on metal ferrocyanides
Adsorption of DL-histidine, DL-threonine and DL-valine on platinum and tungsten ferrocyanides were studied at pH 7.0 ± 0.01 and room temperature 30 ±1(C. Solution pH was maintained by using appropriate buffer mixtures. Adsorption isotherm as C(initial adsorbate concentration) versus q (amount adsorbed mg/g) for adsorption of DL-histidine, DL-threonine, DL-valine on platinum and tungsten ferrocyanides are shown in Figure 2. In general, the adsorption curves are characterized by a gradual rise and flattering at higher adsorbate concentrations. Platinum ferrocyanide was found to have maximum uptake with all three adsorbates (DL-histidine, DL-threonine, DL-valine) in comparison to tungsten ferrocyanide. The values of maximum uptake of amino acids on metal ferrocyanides are given in Table 5.
Table 5 Maximum uptake of amino acids on metal ferrocyanides. Temperature = 30 ± 1(C; pH = 7.0 ± 0.01
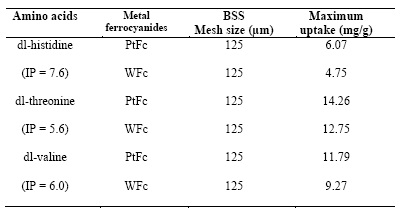
The results obtained on the adsorption of DL-histidine, DL-threonine, DL-valine were analyzed by the models given by Langmuir. The Langmuir Isotherm has been used by various workers for the sorption of a variety of compounds. The model assumes uniform energies of adsorption on to the surface and no transmigration of adsorbate in the plane of the surface. The linear form of Langmuir isotherm is given by following equation(25).
where Qeq is the amount of solute adsorbed per unit mass of adsorbent. Ceq is the equilibrium concentration of solute in solution. Q0 is limiting amount of adsorbate that can be taken up by unit mass of adsorbent. The ‘b’ is constant related to equilibrium constant of bonding energy or enthalpy ((H) of adsorption ( b α e- (H/ RT the parameter ‘b ‘ reflects the steepness of the approach to saturation; more precisely . the ‘b’ value is the reciprocal of concentration at which half of the saturation of adsorbent is attained). Actually, ‘b’ is a constant which is function of adsorption energy. The appropriate Langmuir constants b and Q0 calculated from the slope and intercept of the Langmuir plots, respectively.
When 1/Qeq were plotted against 1/Ceq, straight lines were obtained (Figure 3), which shows that the absorption of DL-histidine, DL-threonine, DL-valine followed the Langmuir isotherm. The Langmuir constants, b and Q0, are calculated and the values are given in Table 6.
Table 6 Langmuir constants and thermodynamic parameter for adsorption of amino acids on metal ferrocyanides Temperature = 30 ± 1(C ; pH = 7.0 ± 0.01; (max dl-histidine = 210 nm; (max dl-threonine = 190 nm; (max dl-valine = 190 nm
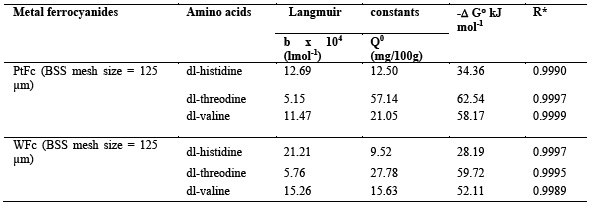
The Q0 values are found to be maximum for PtFc - DL-threonine system, while it is a minimum for the WFC - DL-histidine system. The b values are found to be maximum for the WFC - DL-histidine system and a minimum for the PtFC - DL-threonine system.
The Gibbs free energy for the systems have been calculated using the equation
Where K is the equilibrium constant at temperature T and R is gas universal constant. The calculated values of Gibbs free energy are given in Table 6. The negative Gibbs free energy value indicate the feasibility of the process and spontaneous nature of adsorption.
The correlation co-efficient (R) values are calculated by regression analysis and given in Figure 3. The overall statistics are found to be excellent with an average correlation coefficient of 0.9995. The average standard deviation for the adsorption of DL-histidine, DL-threonine, DL-valine on metal ferrocyanides is found to be 0.0248.
CONCLUDING REMARKS
Platinum and tungsten ferrocyanides were synthesized and utilized for the uptake of amino acids from aqueous solution. Platinum and tungsten ferrocyanides were found to have maximum and minimum adsorption, respectively for all three adsorbates. The high correlation coefficient values indicate high affinity between the metal ferrocyanides surface and amino acids. The research work has shown that metal ferrocyanides to be reasonable and plausible candidate in studies involving adsorption and condensation of biologically important molecules. It can also be concluded from present studies that in a primeval sea experiencing a fluctuating environment (Wetting - drying cycle) as proposed by Lahav and Chang26, metal ferrocyanides could have acted as active surface for concentrating biomolecules.
EXPERIMENTAL
Chemicals
All chemicals reagents used were of analytical - reagent grade. Potassium ferrocyanide, platinune (II) chloride, sodium tungstate, were obtained from BDH, Poole, UK. DL-histidine, DL-threonine and DL-valine were obtained from Aldrich chemicals Co., USA. All chemicals used as such without further purification. All solutions were prepared in double distilled water.
Instruments
A pH meter (Thermo Orion 420 A+) was used for pH measurements. Uvikon spectrophotometer (922, Kontron instruments) was used for spectrophotometric work. Atomic absorption spectrophotometer (Spectra AA - 5, Varian Techtron. Private Ltd.) and CEST-118 CHN analyzer were used for elemental analysis. Infrared spectral data were recorded using the IR - 408 infrared spectrophotometer (Shimadzu) using the paste method.
Synthesis of metal ferrocyanides
Platinum and tungsten ferrocyanides were prepared by Kourim’s method(27). Platinum ferrocyanides was prepared by adding ethyl alcohol to the mixture containing 167 ml of 0.1 M potassium ferrocyanide and 500 ml 0.1 M platinum (II) chloride. While tungsten ferrocyanide was prepared by slowly adding 167 ml 0.1 M potassium ferrocyanide solution to 500 ml, 0.1 M sodium tungstate with constant stirring. The reaction mixtures in both cases were heated on a water bath for 3 h at 60(C with occasional stirring and after cooling was kept at room temperature for 24 h. The precipitate was filtered under vacuum, washed with water and dried in an air oven at 60(C. The dried product was ground and sieved to 125 µm BSS mesh size.
Characterization of metal ferrocyanides
Platinum and tungsten ferrocyanides are found to have dark blue and dark green colour, respectively. The complexes are found to be amorphous solid and shows no X-ray pattern. Both metal ferrocyanides were characterized on the basis of elemental analysis and spectral studies. Platinum, tungsten and iron were estimated by atomic absorption spectrophotometer. Carbon, hydrogen and nitrogen analysis was performed on CEST - 118, CHN analyzer. Percentage composition of elements found in metal ferrocyanides are given in Table 1.
Infrared spectra of the metal ferrocyanides were recorded in KBr disc on Beckman IR- 20 spectrophotometer. A broad band at 3500 cm-1 in both metal ferrocyanides is due to interstitial water molecule and OH group, while the characteristics HOH bending appears at around 1600 cm-1 in both complexes two sharp bands at around 2000 cm-1 and 600 cm-1 are characteristic of cyanide and Fe-C stretching, respectively. Another sharp band at around 500 cm-1 in both metal ferrocyanides probable shows the presence of metal - nitrogen band due to polymerization. All these values are given in Table 2.
Stability of metal ferrocyanides
Both metal ferrocyanides are found to be stable in presence of heat up to 150(C. Above 150(C change in colour may be due to loss of water molecules. Both metal ferrocyanides are found to be stable in acids (HCl, HNO3, H2SO4, CH3COOH) and bases (NaOH, KOH, NH4OH) in concentration range 0.1 - 2.0 M. Metal ferrocyanides are also found to be stable in salt (NaCl, KCl, LiCl, NH4Cl, RbCl, CsCl, BaCl2, CaCl2, MgCl2) solutions in the concentration range 1.0 - 2.0 M.
Adsorption studies
Effect of pH on adsorption of amino acids on metal ferrocyanides
Buffers used were 0.2 M potassium chloride and 0.2 M hydrochloric acid for pH 1.0 - 2.0, 0.2 M acetic acid and 0.2 M sodium acetate for pH 3.0 - 8.0, 0.2 M borax and 0.2 M hydrochloric acid for pH 9.0 and 0.2 M borax and 0.2 M sodium hydroxide for pH 10.0. The pH of such solution was verified using a pH meter (Thermo Orion 420 A+). It was found that species of buffers used do not adsorb on metal ferrocyanides. This was checked by conductivity measurement.
The adsorption of amino acids on metal ferrocyanides at pH range (1.0 - 10.0) and 1.0 x 10-4 M adsorbate concentration was studied. A 10 ml of buffered amino acids solution were added to 100 mg of metal ferrocyanide and placed on a shaker at room temperature (30 ± 1(C) for 1 h, with an additional shaking at half hour interval for 5 h and then centrifuged. The supernatant was decanted and the amino acid concentration determined using the Uvikon spectrophotometer. The concentration of DL-histidine, DL-threonine and DL-valine were measured spectrophotometrically at 210 nm, 190 nm and 190 nm, respectively. The amount of amino acids adsorbed were calculated by the difference in concentration before and after the adsorption.
Effect of concentration on adsorption of amino acids on metal ferrocyanides
The adsorption of DL-histidine, DL-threonine and DL-valine on platinum and tungsten ferrocyanides as a function of their concentration (10-3 - 10-4 M) was studied at pH 7.0 ± 0.01 and room temperature 30 ± 1(C. A series of 15 ml test tubes were employed for the adsorption studies. A 10 ml of buffered amino acid solution was added into test tubes containing 100 mg of metal ferrocyanides and placed on a shaker for 1 h, centrifuged after 6 h with shaking at half hour intervals. The supernatant liquid was decanted and pH of the solution was again noted on pH meter and it was found to be unchanged. The absorbance of DL-histidine, DL-threonine and DL-valine was measured spectrophotometrically at 210 nm, 190 nm, 190 nm respectively. The amount of amino acids adsorbed were calculated by the difference in concentration before and after adsorption (see Table 3 and Table 4) The neutral pH was chosen to investigate the adsorption of amino acids in a wide concentration range because the neutral pH is physiologically significant and most of the redox reactions in biological systems take place in a neutral medium.
Table 3 Percentage Adsorption of dl-histidine, dl-threonine and dl-valine on platinum ferrocyanide at temperature 30 ± 1(C
